Quantum mechanics is "not" a kind of magic
Even scientists don't know yet how to interpret all the results of QM, but we can say for sure what QM is not: some kind of magic.
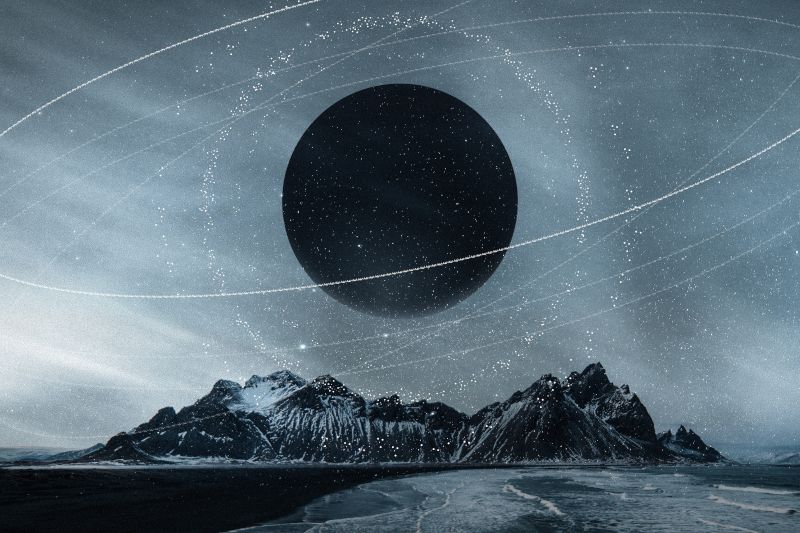
Lately, I have the feeling that, in people's minds, quantum mechanics (QM) is becoming some kind of mysterious current produced by a magical cosmic world. I even read that somebody connected QM with the link between individuals' life and constellations.
Huh?
And while I am perplexed by much of what I read and find around about it, it doesn't seem all that unusual that such a peculiar subject would arouse so much amazement and lead to such interpretations that are at odds with the true nature of the data that physics has given us since the beginning, when QM came out in the last century.
Okay, we can use it to write narratives, stories, and novels but QM is real, not imaginary, and it is not magical, it is physical and it obeys precise laws of physics. Well, precise, maybe we can argue about that. Not because they aren't precise, but because in order to understand how precision is expressed in the quantum view of the world, it is necessary to change the perspective on some things.
A different view
My wife often says to me, "But how can you read essays on quantum physics and understand them?"
Actually, I don't know how much I really understand what I'm studying about QM, but I'm fascinated by it and it pulls me into the subject and pushes me further and further. My approach is that of a curious reader, like when I read a science fiction novel and don't stop until I understand where the various hypotheses on which the plot is based are going to take me. I don't think it's impossible to understand the physics of the subatomic world (because that's what we're talking about), but sometimes it's scary. Because it's obvious that there's still a lot we don't know, and in what we don't know lurk concepts that we find disturbing. Here I would call QM unsettling in the sense that to understand it you have to have the courage to give up certain standard (sometimes comforting) views of reality.
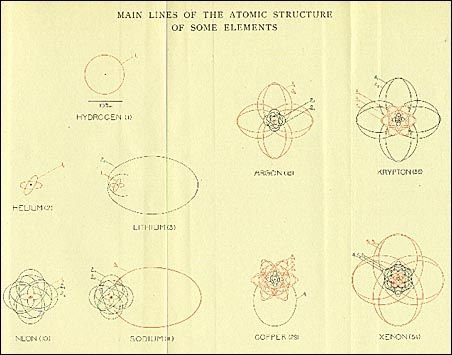
If I want to understand myself better
The subject of QM is the world, the real world, not the imaginary, not secret and hidden vibrations, not just the boundaries of the universe. Its "laws" are hidden deep inside, but it is possible to see them acting on the surface of reality (not only cosmic): semiconductors, transistors, microchips, lasers, computer memories are all things that rely on QM to work. Chemistry is applied QM, so, to our surprise, we ourselves are applied QM; this is one of the reasons why I like to study it. I want to understand myself better, how I process. I want to understand others better, how they exist. I am not talking about mystical, mysterious connections, but dynamics related to specific, quantifiable, explainable functions. QM is not the circus of the impossible; it is the atomic reality around us. And we are atoms, albeit extraordinarily bound together.
Close your eyes
How far away is QM? Where is it? At the edge of the universe? One step away from the event horizon of a big black hole? No, it is within us.
Close your eyes for a few seconds.
I mean really close your eyes.
Are you back? Of course, otherwise you would not be reading. What did you see with your eyes closed? Yes, that's right, "see," because you see something even when you close your eyes. You can see the darkness, and it is never absolute; there are light emissions that have a wavelength just beyond the spectrum of visible light. These infrared rays are emitted by warm bodies all the time. Even from our own bodies. Even from our eyeballs. So, just for a moment, if we could "see" this infrared light, we would be blinded by it, but that does not happen. How come? Because of QM. The photoreceptors in our eyes are sensitive to visible light, but not to infrared light. The way our eye works (and we are still experimenting to understand how) follows QM, the rules of photons. It is photons that hit the retina, and it is possible to observe them by describing their quantum state through a "wave function", a mathematical abstraction that mathematically describes the wave properties of a particle. The fact is that all this is happening in our eyes, not in some galaxy far, far away.
Classical physics VS Quantum physics
We use QM to design new technologies, but also to invent science fiction and fantasy scenarios, but it is not magic, it is science. It is only the assumption on which it is based that challenges the way in which we have always looked at reality, which for us is supposed to be made of solid Newtonian certainty. According to classical physics (CP), the state of a particle can always be defined by its position and velocity. In QM, however, this indispensable certainty (indispensable for us and our perception of reality, not for the nature of things) is replaced by a "cloud of probability".
This means: We cannot know where the particle is we only have probabilities to find out. The closer we get to the nucleus of an atom, the denser this cloud of probability becomes; the farther we go, the more this cloud of probability thins out. This cloud is exactly what we call a "wave function." This is the mathematical abstraction we mentioned earlier. So in CP we have our reassuring "measurements". In QM we have less reassuring "probabilities".
Whenever we look at the world through the classical setting of the system, we set a position and a velocity, and the system evolves according to Newtonian laws of dynamics. On the other hand, when we set the system up quantum, we set up a wave function (a cloud of possibilities) and evolve the system according to the parameters of Schrodinger's equation. Schrodinger's equation tells us that the rate of change of a wave function is proportional to the energy of the quantum system.
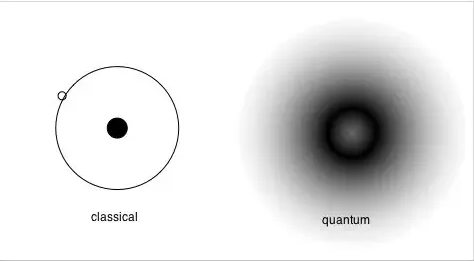
To measure or not to measure? This is the dilemma
So we are talking about energy, and this is logical, considering that a "quanta" is exactly a package with a given energy charge. But avoiding the mathematical details and sticking to the concepts, QM tells us that when we try to make a measurement in a quantum system (remember that we have no fixed speed and position), the probability of getting a certain result is obtained by the wave function, which collapses at the moment of measurement. This is exactly one of the main challenges of QM: measurement, but there is nothing mysterious about it; there are no hidden variables (or are there?). When we measure, the wave function collapses by focusing on one result, and whatever probabilistic distribution it had before the measurement focuses on that single result.
Entanglement state
Logically speaking, in a quantum system, reality is described by a wave function, which is a superposition of all possible occurrences of a given observation. Now, in a quantum system, both the particle (e.g. the electron around the nucleus of an atom) and the measuring device are part of the system, or rather, there is a connection between the two that makes the electron was at a certain position and the measuring device observed it at that exact position (it did not place it first by fixing speed and position, we are in a wave function, thus a probability cloud). When the measurement takes place, the probability cloud collapses at the point where the electron and the instrument are correlated: Entanglement. They "condition" each other. When there is no entanglement, the instrument has not yet observed the electron, but this initial state will evolve into entanglement as soon as the instrument begins to measure and the wave function collapses into a result. If there is no entanglement, the electron is in a "superposition", i.e. in all possible positions, the measurement leads to the entangled state, i.e. a superposition of positions where the instrument could have observed the electron (do you know the cat in the box?). In other words, the electron will only be where the observer could observe it, and that is the entangled state.
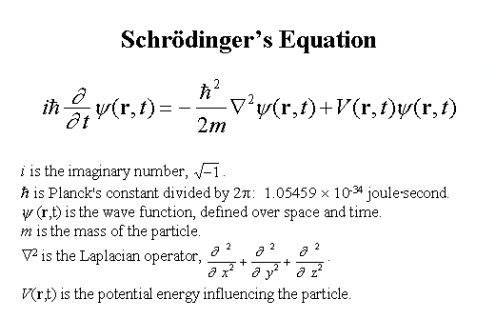
Creativity, hypothesis, not magic
What does all this lead to? Many things, some of them extraordinary. Like Everett's many-worlds theory (instead of a single observer, many observers, each of them connected to a different probable location of the electron and thus located in different worlds). But also to things that are closer to us, such as our own eyeballs. If CP tells us that the world is made of particles and force fields (gravitational, magnetic, electromagnetic), QM tells us that the world is made of a quantum wave function (unique to the whole universe, according to some physicists). Even scientists don't know yet how to interpret all the results of QM, and what it deeply is, but we can say for sure what QM is not: some kind of magic.
Bibliography
- Sean Carroll. (2019). "Something Deeply Hidden: Quantum Worlds and the Emergence of Spacetime". Dutton; Illustrated edition.
- David J. Griffiths and Darrell F. Schroeter. (2018). "Introduction to Quantum Mechanics". Cambridge University Press.
- Nouredine Zettili. (2022). "Quantum Mechanics: Concepts and Applications." Wiley.
- R. Shankar. (1994). "Principles of Quantum Mechanics, 2nd Edition." Plenum Press.
- Andrew J. Larkoski. (2022). "Quantum Mechanics: A Mathematical Introduction." Cambridge University Press.